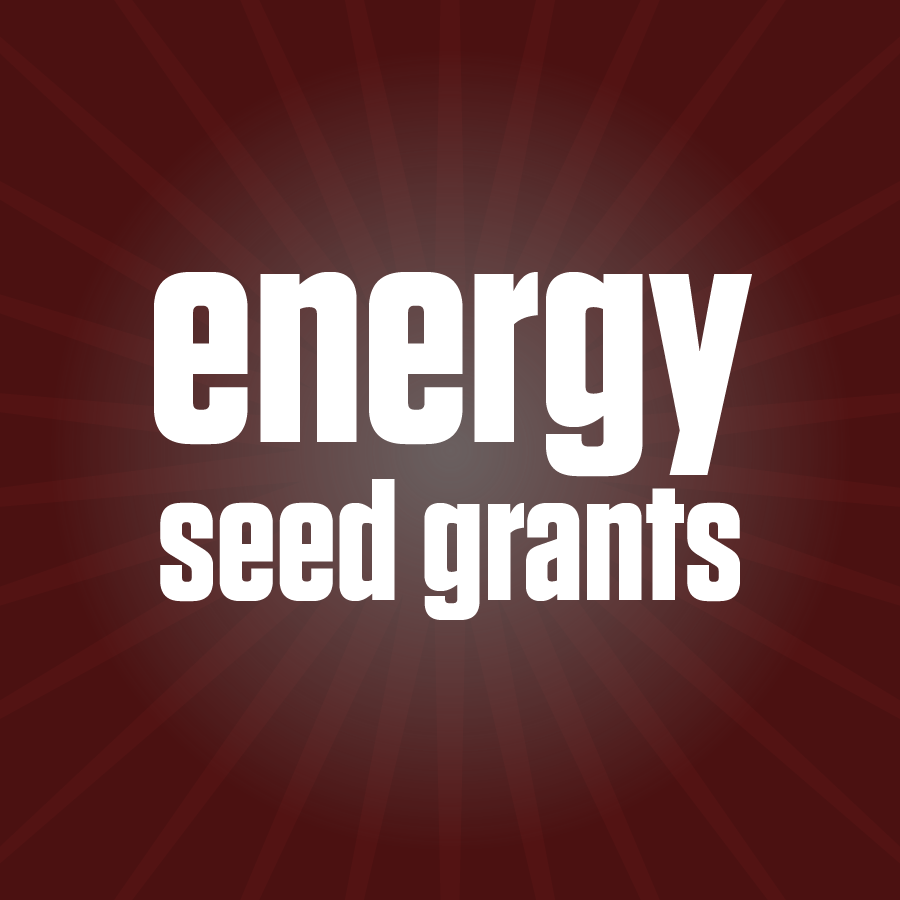
The Texas A&M Energy institute is proud to announce that nine proposals have been selected for funding under its Third Annual “Energy Seed Grant” Research Proposal Call. A total of 38 proposals were submitted with investigators from five colleges at Texas A&M University as well as one college at Texas A&M University-Kingsville.
In its third year, the internal competitive proposal competition has the primary objectives of:
- Encouraging innovative interdisciplinary energy research collaborations.
- Supporting groups of researchers working on important energy-related topics.
The awarded funds will be used for the preparation of competitive group proposals to be submitted to government agencies within 6-12 months of the award. The Third Annual Research Proposal Call submissions were reviewed by the Texas A&M Energy Institute’s faculty steering committee.
2017 Funded Proposals
Third Annual Research Proposal Call
Carboxylate and Thermal Conversion Technology Platforms – Conversion of Biomass to Hydrocarbon Fuels and Designer Biochar
Principal Investigator
Sergio C. Capareda
Professor, Department of Biological and Agricultural Engineering
Co-Principal Investigator
Mark Holtzapple
Professor, Artie McFerrin Department of Chemical Engineering
The carboxylate platform converts biomass wastes to hydrocarbon fuels using a combination of biological and chemical processes. The biological processes employ mixed cultures of microorganisms that convert nearly all biomass components to carboxylic acids without adding external enzymes or using sterile processing conditions. The chemical processes convert carboxylic acids to hydrocarbon fuels with an estimated selling price of $1.00 to $2.50/gal, depending upon the scenario. Hydrogen is a critical feedstock in the process. The focus of the proposed project is to integrate hydrogen derived from the gasification of undigested sludge residues; thus, the entire biomass feedstock is utilized in an optimal manner. In addition, we will explore a new version of the carboxylate platform that recovers the carboxylate salts from the fermentation broth via extraction rather than evaporation, which we have traditionally employed. This new extraction technology improves when the carboxylate salts have a high molecular weight, which is aided by introducing the reducing power of hydrogen directly into the fermentor to elongate the carbon chains. The data produced by this cooperative effort will create a compelling case for attracting funding from both industry and the federal government.
New Model and Data Reduction Strategies Applied to Full Waveform Inversion of Seismic Data
Principal Investigator
Eduardo Gildin
Associate Professor; CJ Craft Faculty Fellow; Harold Vance Department of Petroleum Engineering
Co-Principal Investigators
Richard L. Gibson, Jr.
Francesco Paolo di Gangi/Heep Endowed Professorship and Professor; Department of Geology & Geophysics
Yalchin Efendiev
Professor and Mobil Chair in Computational Science, Department of Mathematics
Nurul Kabir
Adjunct Professor, Department of Geology and Geophysics
Michael King
Professor, LeSuer Chair in Reservoir Management, Harold Vance Department of Petroleum Engineering
Full waveform inversion (FWI) of seismic data encounters problems both because of the complexity of the objective functions that must be minimized and because of the extremely large data and model parameter volumes that are utilized. The presence of many local minima leads to “cycle skipping”, and this problem is often addressed by using low-frequency data in the inversion. However, many conventional algorithms still aim to update model parameters on a finely sampled grid utilized for numerical solutions of the forward wave propagation problem. We propose to explore alternative approaches to model parameterization that we have recently developed and applied in other domains, as well as seismic inversion. Specifically, instead of using a regular discretization of the model parameters such as velocity, we will represent the values using generalized polynomial expressions (and, in the general case, orthogonal function approximations). This is a natural approach for the inversion applied at lower frequencies since the results are smoothly varying fields. Since only a few coefficients are required to specify the velocity field (e.g., polynomial coefficients) instead of a finely sampled set of discrete values, there is a large reduction in the model space with potential for accelerating and simplifying computations. We anticipate that this new approach will lead to a fast and robust reservoir characterization utilizing FWI with far reaching applications in geophysical and subsurface modeling.
A versatile in-situ 3D particle characterization system for combustion, propulsion and energetics applications
Principal Investigator
Waruna Kulatilaka
Associate Professor, Department of Mechanical Engineering
Co-Principal Investigators
Chad Mashuga
Assistant Professor; Artie McFerrin Department of Chemical Engineering
Eric Petersen
Nelson-Jackson Professor, Department of Mechanical Engineering
We propose the development of an advanced, optical-based, portable platform for digitally recording the position, size, and velocity of particles suspended in the gas phase in a variety of engineering applications. In particular, four-dimensional (4D, three spatial dimensions and time) characterization of solid and liquid particles of size ranging from ~10–1000 μm is important in applications such as combustion of solid propellants, diesel sprays, aerosol and soot studies, dust explosions and process safety applications. The proposed scheme is based on recent advances in digital in-line holography (DIH) and ultra-high-frame-rate (up to 1-MHz) imaging cameras. Depth-resolved particle diameter, velocity, and number density will be obtained using sophisticated data processing code developed and available from our research partners at the Sandia National Laboratories. The near-term objective is to demonstrate the proposed 4D DIH system in an existing experimental configuration involving metalized solid propellant combustion, thereby to generate critical validation data sets for a research proposal being prepared for a DoD agency in next 6–12- month period. In addition, the applicability of this system will also be demonstrated in existing experimental configurations such as pre-combustion and atomization studies of synthetic jet fuel sprays, dust explosions in industrial processes such as grain factories and coal mines, as well as hybrid rocket motor testing.
Solar-Driven Membrane Distillation for Petrochemical Wastewater Treatment and Reuse
Principal Investigator
Ying Li
Pioneer Natural Resources Faculty Fellow III, Associate Professor, Department of Mechanical Engineering
Co-Principal Investigator
Bill Batchelor
Senior Professor, Zachry Department of Civil Engineering
This is a multidisciplinary collaborative research project that involves mechanical engineering, environmental engineering, and materials science to develop an innovative wastewater treatment and reuse technology for petrochemical industry. This is a novel engineering idea that aims to solve the water shortage challenge faced in the petrochemical industry by utilizing the abundant solar energy available in Texas to treat and recycle water. A total of 104 projects for new or expanded petrochemical plants are currently planned in Texas with total capital costs of $51.3 billion and these plants will demand huge amounts of water, accounting for 37% of the projected water demand for manufacturing throughout the entire state in 2020. The objective of proposed research is to solve this challenge through the development and validation of a conceptual engineering system by the following approaches: (1) design and build an innovative system of wastewater treatment and reuse by coupling membrane distillation with a photoreactor pretreatment, where the pretreatment step will degrade organic pollutants and heat up feed water driven by sunlight; (2) understand petrochemical wastewater characteristics and water reuse regulations for petrochemical industry; and (3) measure the performance of solar-driven membrane distillation and evaluate the feasibility of the proposed technology for petrochemical wastewater treatment and reuse. The main outcome of the project will be a prototype of the proposed solar-driven membrane distillation system and preliminary research results that can be used for follow-up external grant proposals. The findings from the proposed research will advance the fundamental science in materials and water chemistry and will have potentially significant impacts on the development of both fossil (petrochemical) energy and renewable (solar) energy technologies.
Quantum dot-enabled concentrating solar spectral converters
Principal Investigator
Christi K. Madsen
Professor, Department of Electrical & Computer Engineering
Co-Principal Investigators
Matthew Sheldon
Assistant Professor, Department of Chemistry
Shiren Wang
Associate Professor, Department of Industrial & Systems Engineering
Given the challenge in achieving photovoltaic efficiencies nearing 50% with costly multi-junction photovoltaic cells due to the broadband nature of the solar spectrum, we propose to address efficient spectral converters as a path to novel concentrating luminescent converters. We have formed a research team composed of Professors Christi Madsen (Electrical and Computer Engineering), Matt Sheldon (Chemistry) and Shiren Wang (Industrial Engineering). Based on new materials from the Sheldon group with “unprecedented quantum fluorescence yield,” we will explore ways to integrate the materials into efficient optical waveguides and cavities fabricated using femtosecond laser-based glass processing from the Madsen group. In addition, we will explore integrating more traditional chalcogenide QDs with silica (SiO2). Silica is preferred over organics such as acrylics because it can withstand high temperatures and provides negligible absorption loss over the solar spectrum. Thus, novel devices for high concentration, high-efficiency converters should be enabled. The optical properties of as-prepared QD/SiO2 film will be examined, to enable design work for incorporating them into thin-film devices and maximizing their integrated photoluminescent quantum yield.
MXenes as a new family of tailorable 2D materials for energy storage
Principal Investigator
Miladin Radovic
Associate Professor; Associate Department Head of Materials Science & Engineering
Co-Principal Investigators
Micah Green
Associate Professor, Artie McFerrin Department of Chemical Engineering
Jodie Lutkenhaus
Associate Professor, William and Ruth Neely Faculty Fellow, Artie McFerrin Department of Chemical Engineering
MXenes are two-dimensional (2D) early transition metals (M phase) carbides and nitrides that sparked recently significant interests as novel energy storage materials. They can store energy by a capacitive mechanism and have demonstrated capacities on par with that of graphene. However, a key difference is that the capacitance and charge storage may be finely tuned by the atomic composition of the MXene sheets themselves, whereas graphene sheets have less atomic versatility. In addition, MXenes’ capacitance can be tailored by altering their morphology. We hypothesize that this control over atomic composition and morphology may generate improved capacitance or rate capability in a manner similar to improvements observed with graphene sheets. Here we propose to produce electrodes from MXenes with different composition and morphology and characterize their capacitance, conductivity, as well as power and rate capability. Fundamental scientific insights generated by Texas A&M’s multidisciplinary team in this project will be critical for seeking further funding on designing composition, morphology, and assembly of MXene-based pseudocapacitors.
Coupled dynamics of methane bubble dissolution and hydrate formation
Principal Investigator
Scott Socolofsky
Professor; Division Head, Environmental, Water Resources & Coastal Engineering; Zachry Department of Civil Engineering
Co-Principal Investigators
Sungyon Lee
Assistant Professor, Department of Mechanical Engineering
Yucel Akkutlu
Associate Professor; George & Joan Voneiff Career Development Professor; Harold Vance Department of Petroleum Engineering
The main objective of this proposal is to build an innovative high-pressure water tunnel in the existing Gas Hydrates Laboratory in Petroleum Engineering at Texas A&M University to experimentally investigate the fundamental mechanisms of methane hydrate formation and bubble dissolution for bubbles rising in the deep ocean water column. By combining the expertise in natural gas seeps (PI Socolofsky) and bubble dynamics (co-PI Lee) with the expertise and existing instrumentation for gas hydrates investigation (co-PI Akkutlu), the interdisciplinary research team will directly address the effects of hydrate formation on methane bubble dissolution. The research outcomes will lead to fundamental understanding of hydrate formation and dissolution dynamics of natural gas hydrates in complex systems, necessary to predict the global methane budget, to design natural gas hydrate extraction methods, and to inform remote sensing platforms searching for natural gas seeps that are correlated with new fossil fuel reservoirs.
Risk-aware planning and human-factor evaluation of water and synthetic power networks
Principal Investigator
Le Xie
Associate Professor, Department of Electrical & Computer Engineering
Co-Principal Investigators
John C. Tracy
Director, Texas Water Resources Institute; Professor, Zachry Department of Civil Engineering
Yu (Yvette) Zhang
Assistant Professor, Department of Agricultural Economics
The US food, energy, and water (FEW) systems contain many points of intersection and overlap. The nexus of these is becoming increasingly important with beneficial opportunities arising to coordinate FEW planning including means to handle risk. A prime example is the 2011 Texas drought where we saw rolling power outages and cities running out of water use. The objective of this seed project is to introduce and test an integrated analytical framework that would transform planning and evaluation of water and electric energy systems. While the scientific community has made great progress on FEW component systems, there is still major room for improvement regarding: (i) development of an open-access, verifiable, network model that contain detailed hypothetical electric grid transmission models, (ii) establishing coordinated planning across the FEW Nexus domains with explicit consideration of risk; and (iii) evaluating cooperation enhancement effectiveness through human factor research.
Retrosynthetic design of metal-organic frameworks for clean energy applications
Principal Investigator
Hong-Cai (Joe) Zhou
Professor; Robert A. Welch Foundation Chair; Department of Chemistry
Co-Principal Investigator
Xiaofeng Qian
Assistant Professor, Department of Materials Science & Engineering
In order to design next-generation solid-state materials for advanced energy technologies such as energy harvesting, conversion, and storage, the placement of matter on an atomic and molecular level with atom and energy efficiency is required. Tailoring the properties of matter to produce materials with new capabilities requires both an understanding of how the molecular structure produces those properties, and an unprecedented ability to design that structure. The over-arching goal of this proposal is, therefore, to demonstrate the feasibility of retrosynthetic design of metal-organic frameworks (MOFs) with the precise control of pore size and shape and the precise placement of multiple different functional groups at tunable distances for targeted clean energy applications via close collaborations between theory and experiment (shown in Figure 1). We will apply these synthetic techniques towards the experimental realization of hypothetical MOF structures to produce optimized MOF-based semiconductors, photocatalytic water splitting, and gas phase catalysts, and to derive key insights about the behavior of matter in fundamentally new configurations. The fundamental breakthrough around which this proposal is centered is our collective ability to synthesize rationally-designed, precisely-customized solid-state materials, as opposed to merely taking advantage of the properties of materials discovered through trial and error. With our contributing team members from both synthetic and computational field, we will achieve success by combining the theoretical and computational design of materials, developing cost-efficient synthetic methods, and applying our synthesized materials to applications that will have an impact in future energy technologies.