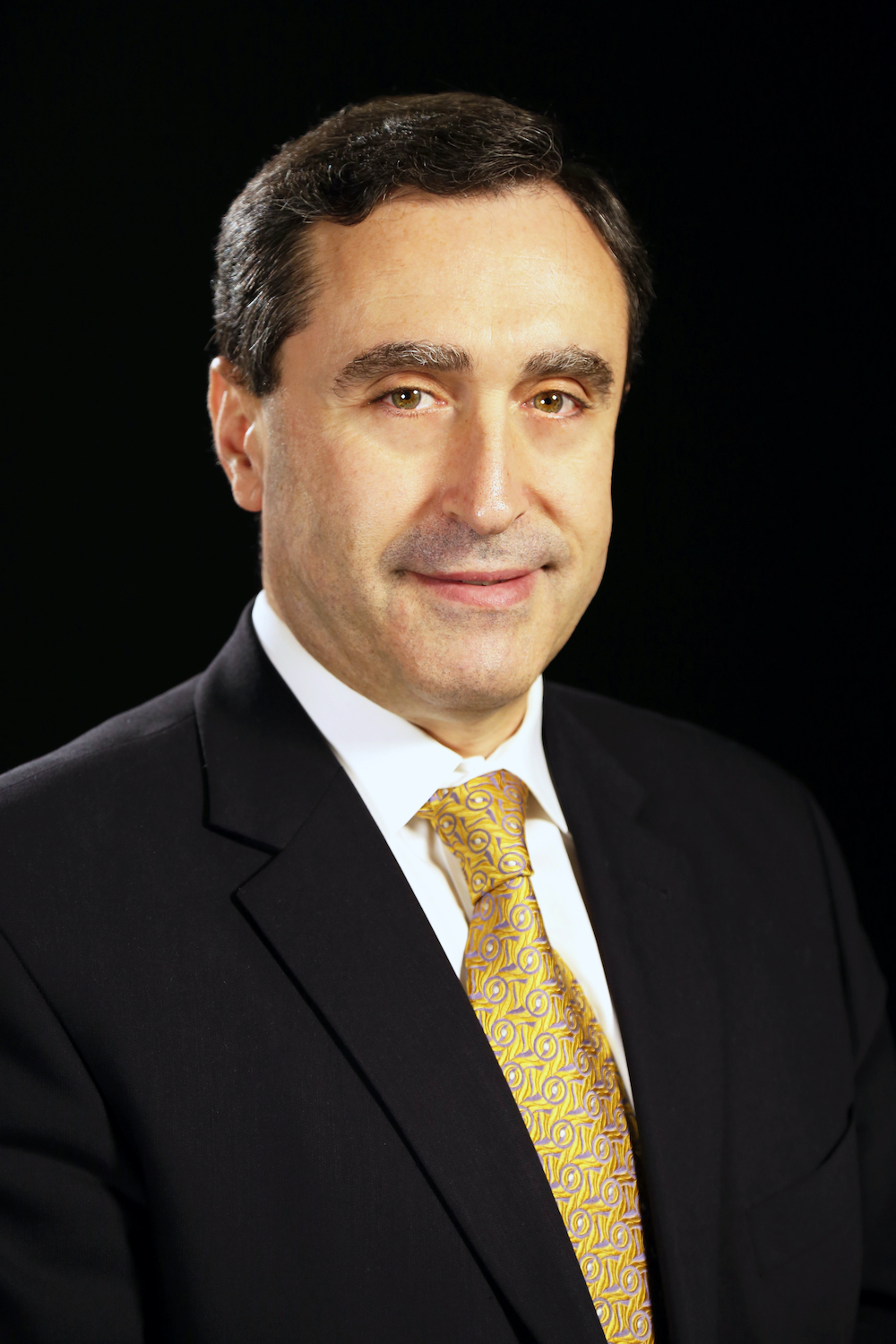
Professor Christodoulos A. Floudas, director of the Texas A&M Energy Institute and the Erle Nye ’59 Chair Professor for Engineering Excellence in the Artie McFerrin Department of Chemical Engineering at Texas A&M University, and his research group have recently published two significant articles on the topics of multi-scale systems engineering and computational prediction of HIV-1 tropism.
Perspective Article: Multi-Scale Systems Engineering is Leading Researchers to an Environmentally Sustainable Energy Economy
“Multi-Scale Systems Engineering for Energy and the Environment: Challenges and Opportunities”
https://aiche.onlinelibrary.wiley.com/doi/abs/10.1002/aic.15151
Multi-scale systems engineering has and will lead researchers to breakthroughs that address challenges related to energy and the environment.
Featured on the cover of the March 2016 issue of the AIChE Journal, Professor Floudas, along with graduate students Alexander M. Niziolek, Onur Onel and Logan R. Matthews, highlight key research areas that multi-scale systems engineering has and continues to address in this perspective article that focuses on CO2 capture, utilization and storage; renewable energy; natural gas-based processes; and production of valuable chemicals.
Within the article, the advances of Texas A&M Energy Institute Faculty Affiliates in applicable areas are referenced, including Professor Ioannis Economou, Professor Mahmoud El-Halwagi, Assistant Professor Faruque Hasan, Professor Mark Holtzapple, Professor Stratos Pistikopoulos, and Associate Professor Benjamin Wilhite.
Global grand challenges facing the energy and environmental sectors are highlighted by governmental, intergovernmental and corporate entities. They hinge on concerns related to energy security, energy affordability and the generation of lower-carbon energy. Multi-scale systems engineering has focused on addressing several of these challenges across scales that vary temporally and/or spatially through the use of principal engineering components: modeling, design, synthesis, simulation and optimization.
The list of influential directions that stem from the emergence of multi-scale systems engineering includes:
- The growth of renewable energy;
- New processes for carbon dioxide capture, utilization and storage; and
- Multi-scale methods for design, simulation and optimization of energy processes, among others.
Computational methods have made it possible to accurately predict properties of CO2 mixtures and design novel materials for CO2 separations, which naturally lends itself to design more efficient carbon capture and sequestration processes. Furthermore, the development of efficient and cost-effective renewable processes has shown the potential to achieve remarkable reductions in greenhouse gas emissions.
The increased focus on natural gas in multi-scale systems engineering has led to significant contributions in methane purification, reactor network design, optimal natural gas conversion and blueprints for the strategic deployment of gas-to-liquids refineries. These contributions are attributed to the rapid development of shale gas reserves in the United States. Finally, in response to the expected future growth of the chemicals industry, multi-scale systems engineering techniques have aided in the development of flexible and selective processes for chemicals production from various feedstocks, such as shale gas.
The tools, frameworks and methodologies developed lay a solid foundation to address the next generation of challenges, which include the food, energy and water nexus; uncertainty quantification in energy systems; and the intermittency of various renewable sources.
Predicting the Path of Cellular Entry for HIV-1
“Highly Accurate Structure-Based Prediction of HIV-1 Coreceptor Usage Suggests Intermolecular Interactions Driving Tropism”
https://doi.org/10.1371/journal.pone.0148974
The human immunodeficiency virus, HIV-1, can infect, via entry, a variety of cells. The specific cell-type infected by a viral strain is referred to as HIV-1 tropism and can affect treatment and overall patient outcome. Cell-based methods for determining HIV-1 tropism are costly with a slow turnaround, and as a result there has been significant interest in developing computational tools for predicting HIV-1 tropism based on isolated DNA sequences encoding the virus.
As described in an article recently published in PLOS ONE, researchers led by Professor Floudas, have made a significant breakthrough in the computational prediction of HIV-1 tropism [1]. The research team responsible for this work includes Assistant Professor Phanourios Tamamis, postdoctoral research associate Dr. Chris A. Kieslich, and doctoral students Yannis Guzman and Melis Onel.
HIV-1 remains difficult to treat due to its high genetic variability. One approach that has been adopted to circumvent the difficulties of thwarting the quickly mutating virus is to target a specific class of human cell-bound proteins, referred to as coreceptors, which are hijacked by HIV-1 for cellular entry. The coreceptor used for cellular entry typically drives HIV-1 tropism, and is based on an interaction with a short segment of a HIV-1 envelope protein. Unlike existing tropism prediction methods, the method developed by Professor Floudas and his team accounts for the specific amino acid interactions between the human and viral proteins that drive coreceptor usage. As a result of this development, the new method provides significant improvements in accuracy in comparison to existing methods. This advance was enabled by computationally-derived complex structures for the interactions between the segment of HIV-1 envelope protein gp120 and human coreceptors, CCR5 [2] and CXCR4 [3], which were also developed by the Floudas group.
The team has also developed the web tool CRUSH (CoReceptor Usage prediction for HIV-1), which is freely available to the academic research community at http://ares.tamu.edu/CRUSH/. The use of features based on the computationally-derived coreceptor complex structures allows CRUSH to be much simpler than existing methods in terms of computation and complexity. CRUSH accuracies are not anchored to a genetic reference sequence, and as a result, CRUSH provides highly accurate predictions across the major geographic and genotypic HIV-1 subtypes. The improved accuracy and generality of CRUSH makes it an ideal candidate for genotypic determination of HIV-1 tropism in clinical settings, which will be the focus of future work.
Floudas is the director of the Texas A&M Energy Institute, professor and holder of the Erle Nye ’59 Chair for Engineering Excellence in the Artie McFerrin Department of Chemical Engineering in the Dwight Look College of Engineering. During a career that spans four decades, Floudas has developed useful tools for optimization of, and found novel pathways for, energy conversion and conservation. The scope of his research includes chemical process synthesis and design, process control and operations, discrete-continuous nonlinear optimization, local and global optimization, and computational chemistry and molecular biology. Floudas is a member of the National Academy of Engineering, the Biophysical Society, the Society of Industrial and Applied Mathematics and the Academy of Athens.
Full Articles:
Floudas, CA, Niziolek, AM, Onel, O, Matthews, LR. Multi-Scale Systems Engineering for Energy and the Environment: Challenges and Opportunities. AIChE Journal 2016, 62 (3), 602-623. doi:10.1002/aic.15151
Kieslich CA, Tamamis P, Guzman YA, Onel M, Floudas CA. Highly Accurate Structure-Based Prediction of HIV-1 Coreceptor Usage Suggests Intermolecular Interactions Driving Tropism. PLoS ONE 11(2): e0148974. 2016. doi:10.1371/journal.pone.0148974
References:
- Kieslich CA, Tamamis P, Guzman YA, Onel M, Floudas CA. Highly Accurate Structure-Based Prediction of HIV-1 Coreceptor Usage Suggests Intermolecular Interactions Driving Tropism. PLoS ONE. Public Library of Science; 2016;11: e0148974. doi:1371/journal.pone.0148974
- Tamamis P, Floudas CA. Molecular Recognition of CCR5 by an HIV-1 gp120 V3 Loop. PLoS ONE. 2014;9. doi:1371/journal.pone.0095767
- Tamamis P, Floudas CA. Molecular Recognition of CXCR4 by a Dual Tropic HIV-1 gp120 V3 Loop. Biophysical Journal. 2013;105: 1502–1514. doi:1016/j.bpj.2013.07.049