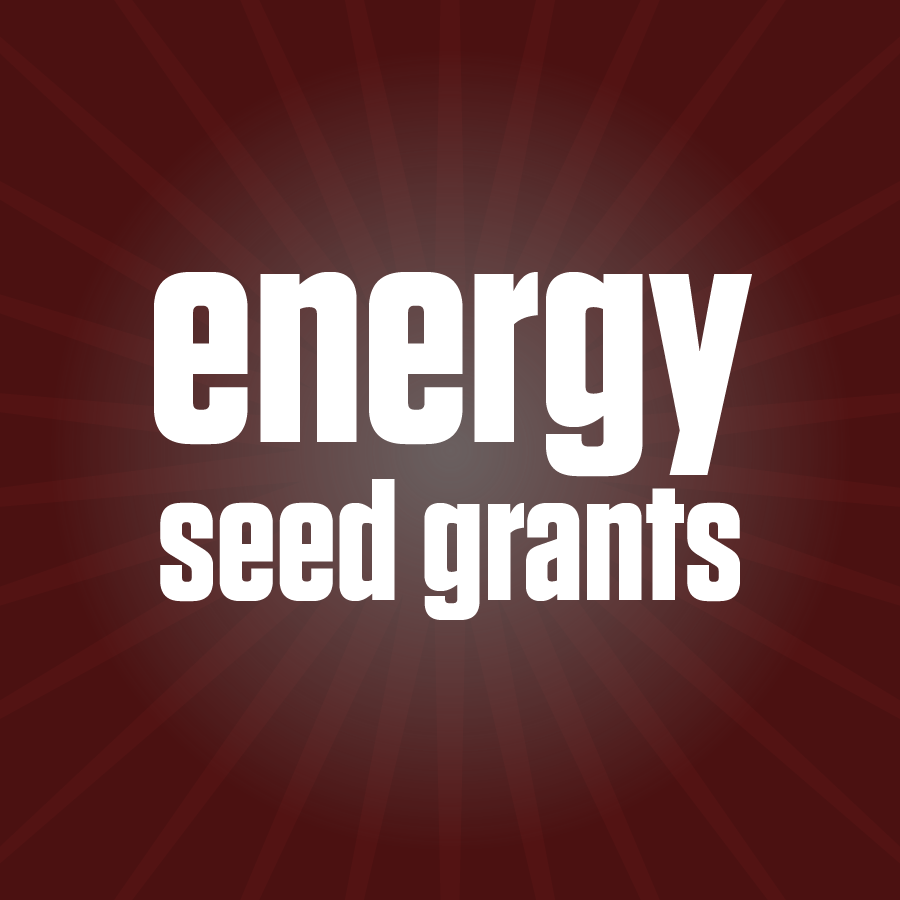
The Texas A&M Energy institute is proud to announce that nine proposals have been selected for funding under its Fourth Annual “Energy Seed Grant” Research Proposal Call. A total of 32 proposals were submitted with investigators from five colleges and 19 departments at Texas A&M University.
In its fourth year, the internal competitive proposal competition has the primary objectives of:
- Encouraging innovative interdisciplinary energy research collaborations.
- Supporting groups of researchers working on important energy-related topics.
The awarded funds will be used for the preparation of competitive group proposals to be submitted to government agencies within 6-12 months of the award. The Fourth Annual Research Proposal Call submissions were reviewed by the Texas A&M Energy Institute’s faculty steering committee and previous Energy Seed Grant investigators.
2018 Funded Proposals
Fourth Annual Research Proposal Call
Fully Encapsulated Autonomous Nuclear Buoy (FEANB) for Distributed and Localized Needs
Principal Investigator
Sunil Chirayath
Associate Professor, Department of Nuclear Engineering
Co-Principal Investigators
Moo-Hyun (Joe) Kim
Professor, Department of Civil Engineering, Department of Ocean Engineering
Lin Shao
Professor, Department of Nuclear Engineering
Pavel Tsvetkov
Associate Professor, Department of Nuclear Engineering
This proposal introduces a novel, scalable, autonomous, multipurpose energy system with a compact scalable micro-modular reactor – Fully Encapsulated Autonomous Nuclear Buoy (FEANB). The FEANB is envisioned as a small floating power complex in which a compact high-efficiency nuclear system provides the source of energy for a variety of industrial processes including electricity, heat, and water in a scalable fully encapsulated robust package within the body of a floating buoy. The FEANB can be used for a variety of applications requiring mobility and high degrees of robustness and resilience to environmental conditions as compared to traditional mobile power systems. The concept is envisioned to be especially suitable for regions and communities where local conditions lead to separation and isolation from common energy grid infrastructures, energy resources, and supplies.
Force Driven Chemical Synthesis of Metal-Organic Frameworks
Principal Investigator
Jonathan Felts
Assistant Professor, Department of Mechanical Engineering
Co-Principal Investigator
James D. Batteas
Professor, Department of Chemistry, Department of Materials Science & Engineering
Metal-organic frameworks (MOFs) are a crucial material within the petroleum and petrochemical industries. Although one of the strengths of the MOF family of materials is the ability to synthesize a wide range of chemical compositions, precisely controlling these compositions is often not straightforward, further increasing complexity and cost. This project seeks to design a radically different mechanochemical reactor that will provide unrivaled control over the forces applied to the reactants, providing a way to rationally explore how force drives the formation of products. This force controlled mechanochemical reactor will allow for long-term exploration of mechanochemical synthesis of MOFs and other products critical to the petroleum and petrochemical industries.
Intraday Trading for Electricity Markets
Principal Investigator
Alfredo Garcia
Professor, Department of Industrial and Systems Engineering
Co-Principal Investigators
Erick Moreno-Centeno
Professor, Department of Industrial and Systems Engineering
Anastasia Shcherbakova
Professor, Department of Agricultural Economics
Reid Stevens
Professor, Department of Agricultural Economics
The increasing penetration of intermittent renewables has flattened supply curves and increased the need for flexible resources that can quickly and reliably respond to time-varying market conditions. In this project, we propose a method for reducing the time inconsistency in power planning: intraday financial settlements. Shortening the financial settlement horizon will allow renewable generators to base their bids on more accurate information, leading to more efficient settlement outcomes. As an initial test of our hypothesis, we propose to conduct a counterfactual study and compare the efficiency of system outcomes. We will base our study on data from the Electric Reliability Council of Texas (ERCOT) for the year 2017.
Efficient and Optimal Control of Solar Modules via Prediction of Supply and Demand Under Real-World Environmental Uncertainties
Principal Investigator
Shima Hajimirza
Assistant Professor, Department of Mechanical Engineering
Co-Principal Investigator
Robert S. Balog
Associate Professor, Department of Electrical & Computer Engineering
Accurate forecasting of solar power generation is a necessary step in stabilizing and securing grid operations that rely on PV systems. The proposed project will design a framework in which combined supply/demand predictions and optimized control can maximize the reliability of grid-connected PV systems. Energy supply is predicted based on environmental factors. Demand is predicted using time-series forecast methods and external factors such as climate conditions. Given supply and demand, control algorithms are proposed to adjust the operating state of the PV system. The goal of such algorithms is to meet the demand in real time, given the supply model with as few adjustments as possible.
Bio-Inspired Design of Complex Energy Systems to Achieve Robust, Efficient, and Sustainable Networks
Principal Investigator
Astrid C. Layton
Assistant Professor, Department of Mechanical Engineering
Co-Principal Investigator
Katherine Davis
Assistant Professor, Department of Electrical & Computer Engineering
The U.S. energy network is made up of hundreds of thousands of high-voltage transmission lines that connect generation sources to loads. This vast network of energy producers and consumers looks analogous to the biological ecosystem representation of a food web, where producer-consumer translates to prey-predator. The proposed work will investigate methods to study these complex energy systems using ecological analysis techniques. In the field of engineering design, ecosystems offer insight into how to model interactions of components, including connectivity and dependencies between components or actors as well as resources and their flows. In this work, the goal is to discover how techniques from ecological analysis and ecosystem behavior may provide insight into new methods and guidelines for enhancing the resilience and robustness of energy networks for both planning and operations while considering realistic growth patterns of load and generation, including sustainable energy sources that may reach into the distribution level.
Extreme Weather and Gulf Coast Energy Systems: Present and Future Threats, Vulnerabilities and Path to Adaptation
Principal Investigator
Bruce A. McCarl
University Distinguished Professor, Department of Agricultural Economics
Co-Principal Investigator
Yangyang Xu
Assistant Professor, Department of Atmospheric Sciences
The Texas Gulf Coast is the home to approximately $800 billion worth of energy assets. Recent disasters have shown that such assets are vulnerable to extreme weather events. Research-based information is greatly needed on the magnitude of the threat, the extent of the vulnerability and the types of adaptation strategies that are being deployed or could be employed. This project’s proposed efforts will involve: a) estimating the localized nature of both current and projected threats through high-resolution climate modeling, b) estimating the economic consequences to the energy industry (extraction, processing, generation, transmission and associated transport) as realized so far and as projected into the future, and c) doing a preliminary survey of possible adaptation strategies including where possible an implementation cost estimate, and a back of the envelope economic evaluation of major adaptation possibilities.
Scalable Microfluidic-based Ion Concentration Polarization Desalination for Recycling/Disposal of Produced and Flow Back Water in Shale Oil/Gas Development
Principal Investigator
Hadi Nasrabadi
Assistant Professor, Department of Petroleum Engineering
Co-Principal Investigator
Debjyoti Banerjee
Professor, Department of Mechanical Engineering
In this project, we will conduct an experimental and modeling study on the feasibility of using a novel desalination technique (Ion Concentration Polarization Desalination) for recycling/disposal of produced and flow back water in shale oil/gas development. To the best of our knowledge, this method has never applied before in treatment of produced/flow back water from shale development. We will fabricate a simple microfluidic device to perform our desalination experiments on produced and flow back water samples. Parallel to experiments, we will use numerical simulation to model the experiments and optimize the performance of this technique for field application. Finally, we will fabricate microfluidic devices based on out-of-plane ion concentration polarization method that can be directly used for scalable produced water treatment.
Strain Modulation of 2D Materials as a Means of Enhancing Electrocatalytic Activity
Principal Investigator
Matt Pharr
Assistant Professor, Department of Mechanical Engineering
Co-Principal Investigators
Sarbajit Banerjee
Professor, Department of Chemistry
Xiaofeng Qian
Assistant Professor, Department of Materials Science & Engineering
Owing to their distinctive electronic, optical, and structural properties, 2D materials have emerged as promising candidates for energy relevant catalysis, including electrocatalysis, photocatalysis, and conventional heterogeneous catalysis. Still, prior to achieving practical applications, 2D catalysts must function at lower overpotentials with higher turnover frequencies and improved product selectivity. As such, fundamental studies are required to provide insight into the key chemistries and physics that produce these desirable characteristics. To this end, the proposed work aims to provide a fundamental understanding of the catalytic nature of 2D materials by in situ characterization and theoretical simulations. In particular, the research team will focus on the effects of mechanical strain on electrocatalysis.
Integration of Physics-based Models and Model-based control algorithms to Improve Productivity in Hydraulic Fracturing Treatments
Principal Investigator
Kan Wu
Assistant Professor, Department of Petroleum Engineering
Co-Principal Investigator
Joseph Sang-II Kwon
Assistant Professor, Department of Chemical Engineering
Unconventional natural gas and oil resources are found in rock formations of ultra-low permeability. What has made the recovery of shale oil economically viable is the extensive use of multiple hydraulic fracturing treatments. In hydraulic fracturing, the proppant-filled fracture length at the end of pumping strongly influences the fluid conductivity of oil and gas; however, proppant cannot be evenly distributed throughout complex fracture networks due to the limited proppant transport of thin fracturing fluids as well as a complex nature of fracture propagation in unconventional reservoirs. Motivated by these considerations, the proposed research will first address the development of a new high-fidelity model to describe the fracture propagation by explicitly accounting for the effect of fracture interactions as well as proppant transport to fluid conductivity. Second, utilizing the new high-fidelity model, we will focus on introducing a new model-based control algorithm to compute online pumping schedules that compensate for the fracture interaction; the computed pumping schedule will be able to generate uniform proppant distribution throughout the entire fracture network.